What Color Is Your Hydrogen?
What Color Is Your Hydrogen?
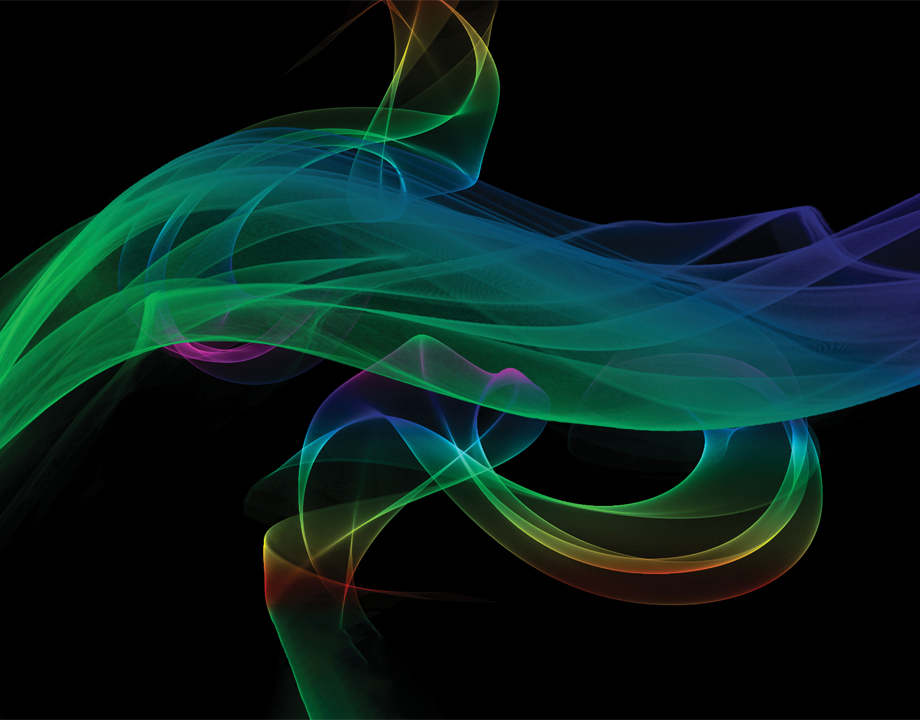
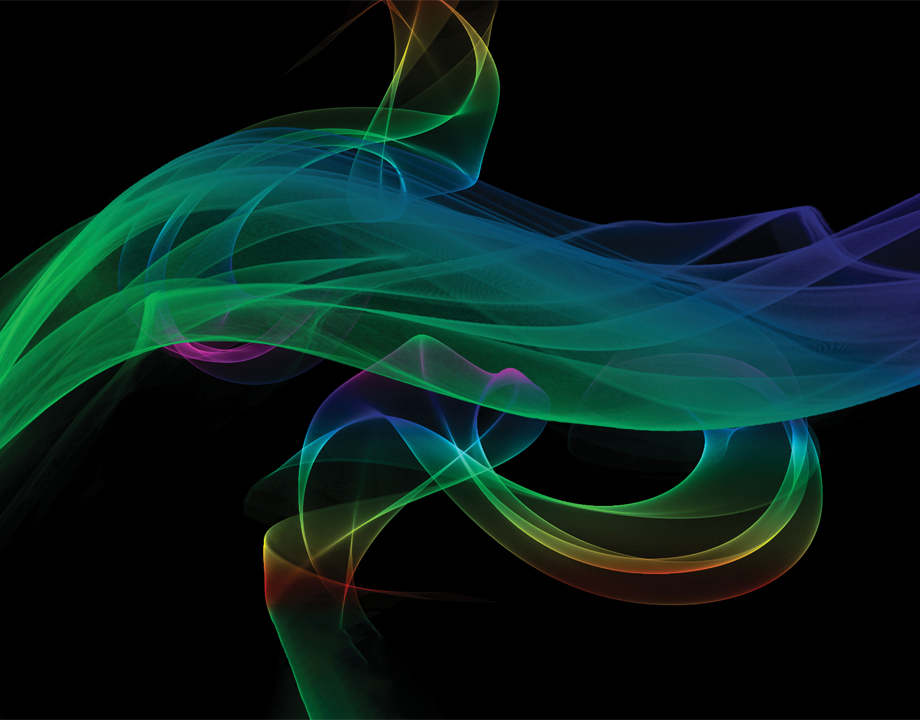
For an odorless, colorless gas, hydrogen has a rainbow of adjectives. Researchers and startups are looking for ways to make it greener. Image: Getty
As wise people from Niels Bohr to Yogi Berra have noted, predictions are hard, especially about the future. But politicians can’t help themselves; they spin the rosiest scenarios about the outcomes of their policy proposals. It would have to be an optimistic leader who declares, “With a new national commitment, our scientists and engineers will overcome obstacles to taking these cars from laboratory to showroom, so that the first car driven by a child born today could be powered by hydrogen, and pollution-free.” But any children born when President George W. Bush made that pronouncement would be 18 years old this year, and few if any of them are driving hydrogen-powered cars.
That might be for the best. In spite of its clean reputation, hydrogen is an energy carrier rather than a source and is only as environmentally friendly as the method for producing it. In 2019, the world used up to 80 million tons of hydrogen, much of it for oil refining and ammonia production, most of it produced via the steam reformation of methane (SRM). The process is relatively inexpensive, but it is energy-intensive and results in not only hydrogen gas but also carbon dioxide, so much so that it is derisively referred to as “gray” hydrogen (or even “brown” hydrogen when the carbon feedstock is coal). Had the Bush administration’s hydrogen proposals taken off, it might have had only a marginal effect on greenhouse gas emissions and energy consumption.
More about Sustainable Engineering: Carbon Reuse Turns a Cost into a Benefit
It’s possible to produce hydrogen in ways that don’t have those carbon impacts, and they are color-coded, too. Capturing the carbon dioxide generated through steam reformation and sequestering is referred to as “blue” hydrogen; If that carbon is converted to a solid form, that, according to DOE, is called “turquoise hydrogen.” But most of the research and entrepreneurial interest is in the “green” part of the spectrum: Processes that do not generate net carbon emissions in the first place.
Keith Wipke, fuel cell and hydrogen technologies program manager at the National Renewable Energy Laboratory, acknowledges that cost plays a big role in whether the greener-hued hydrogen technologies are adopted. “Everybody wants the best environment pathway,” Wipke said, “but that has to include the economics. That’s why our emphasis here at DOE is to dramatically bring down the cost of producing clean hydrogen.”
While steam reforming is a fairly standard process, there is no agreed-upon best path for green hydrogen. The scramble is on to find the least expensive and most energy-efficient method.
Electrolysis—the use of electricity to split water molecules into hydrogen and oxygen—is a staple of high school chemistry classes. It is also one focus of green hydrogen research, since if the electricity is produced through wind, solar, nuclear, or some other non-fossil fuel means, the hydrogen would be considered zero-carbon and could be used as an alternative to batteries for storing renewable energy.
While the electrolytic production of hydrogen was first demonstrated in 1800, the process is still too expensive to be broadly competitive with SRM. There are a few reasons for this. An assessment of efforts to reduce the cost of electrolysis by the International Renewable Energy Association found that the biggest driver is the cost of renewable energy. According to the report, electrolysis could be competitive with SRM at a renewable power cost of $20 per MWh. Given the rapidly falling costs of solar and wind, there is growing optimism that renewable green hydrogen will be economically competitive the steam-reformed hydrogen—and eventually with fossil fuels, which is estimated to occur when hydrogen fuel reaches a price of $2.50 per kilogram.
Research is working on improving the electrolysis process, as well. Attempts to improve electrolyzer performance and efficiency—which presently ranges between 65 percent and 85 percent—have focused on materials for electrodes and catalysts, as well as on the new electrolytes in solid form or dissolved in water to help make it electrically conductive. Another avenue for optimization is in the membranes that allow gases to selectively pass through, enabling the separation of hydrogen from contaminants. And research at the Institute of Fluid Dynamics at the Helmholtz-Zentrum Dresden-Rossendorf in Germany has sought to understand why some hydrogen bubbles reattach themselves to electrodes; reducing that effect would also improve overall efficiency.
Even if those issues are overcome, there are still some downsides to the idea of using renewable electricity to make green hydrogen. For one, the intermittent nature of wind and solar power means that the expensive electrolysis equipment would not be in constant use, which drives up the cost per kg of hydrogen produced. And the transportation of hydrogen gas over long distances is fraught with concerns over leakage, safety, and damage to metal components of the transportation system.
Solving the transportation issue in a way that can better utilize existing infrastructure is the focus of several research efforts.
Some research groups are looking at the use of ammonia as a hydrogen carrier. Ammonia (NH3) is a gas at standard temperature and pressures but can be liquefied without much effort. Liquefying it raises its density by a factor of nearly 900, which means liquid ammonia contains far more hydrogen by volume than compressed hydrogen gas. And it can be transported by pipeline much more economically than hydrogen.
Listen to Our Podcast: The Promise of Hydrogen
The goal, said Ali Jalili, an Australian Research Council DECRA Fellow at the University of New South Wales in Sydney, “is to use renewable energy to produce ammonia as a hydrogen carrier, and then to crack the ammonia when and where the hydrogen is needed.”
That renewable pathway will be needed if ammonia is to be part of a green hydrogen system, because the way the ammonia is produced today is basically an environmental nightmare. The commonly used Haber-Bosch process combines hydrogen obtained through SRM with nitrogen from the air in a high-temperature, high pressure, energy-intensive process. In fact, ammonia production accounts for about 1 percent of the world's total power output, and 2 percent of its greenhouse gas emissions.
Jalili’s group is looking at ways to cut the carbon out of ammonia production, though it hasn’t been easy. “Making ammonia from air and water using renewable energy is difficult due to the low solubility and inertness of the atmospheric nitrogen,” he said.
To get around the innate sluggishness of nitrogen gas, Jalili’s group developed a method to produce NOx from the air through the use of nonthermal plasma. The NOx is far more reactive and soluble in the water, boosting ammonia production significantly and efficiently through electrochemistry. Nonthermal plasma produces high energy electrons locally at room temperature, reducing the energy requirement and making it a good fit for small-scale systems that run on renewable energy.
Jalili believes the process is already economically viable but is still working to improve it further. (In theory, the NOx method could be more than twice as efficient as the Haber-Bosch process.) The group’s ongoing research efforts involve improving NOx generation rates, reactor designs, and scalability. They use copper as an effective catalyst rather than the more expensive noble metals often used in hydrogen electrolyzers, and the process can produce ammonia either as a gas or a liquid, or even ammonium nitrate fertilizer.
Watch the Video: What are Electrofuels?
Another net-zero carbon way to produce green hydrogen is to steam reform biomass rather than fossil fuels. San Francisco-based Charm Industrial is working to convert waste biomass (primarily sawdust and wood waste) into bio-oil using fast pyrolysis, which is an exothermic process. The bio-oil is then reformed into carbon-neutral hydrogen.
“The bio-oil replaces the methane input in a similar reactor to create syngas,” said Charm’s chief technology officer and co-founder, Kelly Hering. The syngas the process produces is used to make hydrogen or can be a feedstock for other chemical processes.
While noting that a recent Department of Energy report found that as much as 1 billion dry tons of biomass could be available for energy use annually, Hering said, “It’s not economical to ship biomass and it’s not economical to ship hydrogen.” Converting the biomass to oil makes it much easier to transport, she said.
Hering and co-founder Peter Reinhardt both worked as engineers in the space industry, where they gained experience optimizing proven technologies through iterative design. They have applied the same approach to the challenge of making green hydrogen. In addition to bio-oil, Charm Industrial is looking at pathways to steam reform ethanol or biomethane from landfills or agricultural sites to produce carbon-neutral, green hydrogen.
Other groups are trying to get biological materials to make hydrogen directly. For instance, microbial electrolysis cells use specialized microbes capable of breaking down biomass combined with a small electric current to produces hydrogen through a fermentation process known as direct hydrogen fermentation. Researchers are looking to combine this process with wastewater treatment to turn human waste into hydrogen.
The electrolyzer architecture also can be enhanced in ways that produce useful byproducts or sidestep the need for electricity. Planetary Hydrogen is a Canadian company that is taking this approach, combining hydrogen production with carbon capture. Its system uses an electrolyzer, but instead of simply using electricity to split water into hydrogen and oxygen, the process adds a mineral salt to the electrolyte that results in the production of a mineral hydroxide. The alkaline compound then removes carbon dioxide from the air “using the ocean as both reaction chamber and storage medium,” said Planetary Hydrogen CEO Mike Kelland. “Ultimately what the process does is produce carbon negative hydrogen.”
While this might require more energy per kg than other methods of producing green hydrogen, the added value of carbon removal can potentially make the price competitive. The process is a bit like direct-air capture of carbon dioxide, but Planetary Hydrogen cannot offer a stream of CO2 for sale or storage. Instead, its product is a carbon-negative hydrogen fuel, which the company expects will be attractive going forward given the growing interest in both clean energy and carbon extraction. The alkaline bicarbonate that results from the seawater carbon removal process also reduces ocean acidification.
Other approaches use heat or light, rather than electricity, to drive the chemical reactions. For instance, a thermochemical process applies high temperature heat from solar concentrators or nuclear reactors to water in the presence of other chemicals such as cerium oxide or copper chloride to split hydrogen from oxygen. The chemicals can be reused in a closed loop system that consumes only water.
Microorganisms such as green microalgae or cyanobacteria can split water into oxygen and hydrogen ions in the presence of sunlight in a process called photobiological water splitting. Researchers believe this approach can potentially be a low-cost, net-zero carbon process utilizing undrinkable water or wastewater, though this needs to be proven outside laboratory settings.
Download an Infographic: Clean Electricity Around the World
Finally, some researchers are trying to optimize photosynthetic hydrogen production by removing the biological limitations. Specialized semiconductors known as photoelectrochemical materials can split water in the presence of sunlight. The materials are similar to those used in photovoltaic cells but have been altered so they can be immersed in an electrolyte, where they facilitate what is called direct solar electrolysis or artificial photosynthesis. The approach, which is still at laboratory scale, can potentially provide a high-efficiency, low temperature process that could leverage the proliferation of solar PV technology to achieve low cost and scale.
According to Wipke, DOE’s NREL lab started out on a photoelectrochemical pathway—and reached efficiencies of 16 percent with that approach—but have now widened its focus to include biological approaches, a high-temperature approach utilizing both heat and electricity from a nuclear power plant, and the current front-runner: a low-temperature, electrolysis system based on proton exchange membranes and a polymer electrolyte similar to those used in fuel cells. They also have a power-to-gas pilot operational in California that utilizes excess renewable power to produce either hydrogen gas through electrolysis or renewable natural gas through a process of bio-methanation that uses microorganisms to add carbon dioxide to the hydrogen, producing renewable natural gas.
For green hydrogen to play a large part in decarbonizing the economy, one or more of these approaches—or something similar—will need a breakthrough on price, efficiency, and scalability.
That’s clearly DOE’s vision. The department’s Hydrogen Energy Earth Shot goal is to reduce the cost of green hydrogen by 80 percent to $1 per kilogram over the next 10 years—and expand its use to more than one-sixth of the United States’s primary energy use.
“This will enable a number of new markets including electric power generation, metals manufacturing, heavy transportation, and energy storage among others,” Wipke said, “where the use of clean hydrogen can add value on emissions reduction, economic and energy resilience fronts while being competitive with their more polluting predecessors.”
R.P. Siegel is a science and technology writer based in Rochester, N.Y.
That might be for the best. In spite of its clean reputation, hydrogen is an energy carrier rather than a source and is only as environmentally friendly as the method for producing it. In 2019, the world used up to 80 million tons of hydrogen, much of it for oil refining and ammonia production, most of it produced via the steam reformation of methane (SRM). The process is relatively inexpensive, but it is energy-intensive and results in not only hydrogen gas but also carbon dioxide, so much so that it is derisively referred to as “gray” hydrogen (or even “brown” hydrogen when the carbon feedstock is coal). Had the Bush administration’s hydrogen proposals taken off, it might have had only a marginal effect on greenhouse gas emissions and energy consumption.
More about Sustainable Engineering: Carbon Reuse Turns a Cost into a Benefit
It’s possible to produce hydrogen in ways that don’t have those carbon impacts, and they are color-coded, too. Capturing the carbon dioxide generated through steam reformation and sequestering is referred to as “blue” hydrogen; If that carbon is converted to a solid form, that, according to DOE, is called “turquoise hydrogen.” But most of the research and entrepreneurial interest is in the “green” part of the spectrum: Processes that do not generate net carbon emissions in the first place.
Keith Wipke, fuel cell and hydrogen technologies program manager at the National Renewable Energy Laboratory, acknowledges that cost plays a big role in whether the greener-hued hydrogen technologies are adopted. “Everybody wants the best environment pathway,” Wipke said, “but that has to include the economics. That’s why our emphasis here at DOE is to dramatically bring down the cost of producing clean hydrogen.”
While steam reforming is a fairly standard process, there is no agreed-upon best path for green hydrogen. The scramble is on to find the least expensive and most energy-efficient method.
It’s Not Easy
Electrolysis—the use of electricity to split water molecules into hydrogen and oxygen—is a staple of high school chemistry classes. It is also one focus of green hydrogen research, since if the electricity is produced through wind, solar, nuclear, or some other non-fossil fuel means, the hydrogen would be considered zero-carbon and could be used as an alternative to batteries for storing renewable energy.
While the electrolytic production of hydrogen was first demonstrated in 1800, the process is still too expensive to be broadly competitive with SRM. There are a few reasons for this. An assessment of efforts to reduce the cost of electrolysis by the International Renewable Energy Association found that the biggest driver is the cost of renewable energy. According to the report, electrolysis could be competitive with SRM at a renewable power cost of $20 per MWh. Given the rapidly falling costs of solar and wind, there is growing optimism that renewable green hydrogen will be economically competitive the steam-reformed hydrogen—and eventually with fossil fuels, which is estimated to occur when hydrogen fuel reaches a price of $2.50 per kilogram.
Research is working on improving the electrolysis process, as well. Attempts to improve electrolyzer performance and efficiency—which presently ranges between 65 percent and 85 percent—have focused on materials for electrodes and catalysts, as well as on the new electrolytes in solid form or dissolved in water to help make it electrically conductive. Another avenue for optimization is in the membranes that allow gases to selectively pass through, enabling the separation of hydrogen from contaminants. And research at the Institute of Fluid Dynamics at the Helmholtz-Zentrum Dresden-Rossendorf in Germany has sought to understand why some hydrogen bubbles reattach themselves to electrodes; reducing that effect would also improve overall efficiency.
Even if those issues are overcome, there are still some downsides to the idea of using renewable electricity to make green hydrogen. For one, the intermittent nature of wind and solar power means that the expensive electrolysis equipment would not be in constant use, which drives up the cost per kg of hydrogen produced. And the transportation of hydrogen gas over long distances is fraught with concerns over leakage, safety, and damage to metal components of the transportation system.
Solving the transportation issue in a way that can better utilize existing infrastructure is the focus of several research efforts.
Some research groups are looking at the use of ammonia as a hydrogen carrier. Ammonia (NH3) is a gas at standard temperature and pressures but can be liquefied without much effort. Liquefying it raises its density by a factor of nearly 900, which means liquid ammonia contains far more hydrogen by volume than compressed hydrogen gas. And it can be transported by pipeline much more economically than hydrogen.
Listen to Our Podcast: The Promise of Hydrogen
The goal, said Ali Jalili, an Australian Research Council DECRA Fellow at the University of New South Wales in Sydney, “is to use renewable energy to produce ammonia as a hydrogen carrier, and then to crack the ammonia when and where the hydrogen is needed.”
That renewable pathway will be needed if ammonia is to be part of a green hydrogen system, because the way the ammonia is produced today is basically an environmental nightmare. The commonly used Haber-Bosch process combines hydrogen obtained through SRM with nitrogen from the air in a high-temperature, high pressure, energy-intensive process. In fact, ammonia production accounts for about 1 percent of the world's total power output, and 2 percent of its greenhouse gas emissions.
Jalili’s group is looking at ways to cut the carbon out of ammonia production, though it hasn’t been easy. “Making ammonia from air and water using renewable energy is difficult due to the low solubility and inertness of the atmospheric nitrogen,” he said.
To get around the innate sluggishness of nitrogen gas, Jalili’s group developed a method to produce NOx from the air through the use of nonthermal plasma. The NOx is far more reactive and soluble in the water, boosting ammonia production significantly and efficiently through electrochemistry. Nonthermal plasma produces high energy electrons locally at room temperature, reducing the energy requirement and making it a good fit for small-scale systems that run on renewable energy.
Jalili believes the process is already economically viable but is still working to improve it further. (In theory, the NOx method could be more than twice as efficient as the Haber-Bosch process.) The group’s ongoing research efforts involve improving NOx generation rates, reactor designs, and scalability. They use copper as an effective catalyst rather than the more expensive noble metals often used in hydrogen electrolyzers, and the process can produce ammonia either as a gas or a liquid, or even ammonium nitrate fertilizer.
Watch the Video: What are Electrofuels?
Another net-zero carbon way to produce green hydrogen is to steam reform biomass rather than fossil fuels. San Francisco-based Charm Industrial is working to convert waste biomass (primarily sawdust and wood waste) into bio-oil using fast pyrolysis, which is an exothermic process. The bio-oil is then reformed into carbon-neutral hydrogen.
“The bio-oil replaces the methane input in a similar reactor to create syngas,” said Charm’s chief technology officer and co-founder, Kelly Hering. The syngas the process produces is used to make hydrogen or can be a feedstock for other chemical processes.
While noting that a recent Department of Energy report found that as much as 1 billion dry tons of biomass could be available for energy use annually, Hering said, “It’s not economical to ship biomass and it’s not economical to ship hydrogen.” Converting the biomass to oil makes it much easier to transport, she said.
Hering and co-founder Peter Reinhardt both worked as engineers in the space industry, where they gained experience optimizing proven technologies through iterative design. They have applied the same approach to the challenge of making green hydrogen. In addition to bio-oil, Charm Industrial is looking at pathways to steam reform ethanol or biomethane from landfills or agricultural sites to produce carbon-neutral, green hydrogen.
Other groups are trying to get biological materials to make hydrogen directly. For instance, microbial electrolysis cells use specialized microbes capable of breaking down biomass combined with a small electric current to produces hydrogen through a fermentation process known as direct hydrogen fermentation. Researchers are looking to combine this process with wastewater treatment to turn human waste into hydrogen.
Heat and Light
The electrolyzer architecture also can be enhanced in ways that produce useful byproducts or sidestep the need for electricity. Planetary Hydrogen is a Canadian company that is taking this approach, combining hydrogen production with carbon capture. Its system uses an electrolyzer, but instead of simply using electricity to split water into hydrogen and oxygen, the process adds a mineral salt to the electrolyte that results in the production of a mineral hydroxide. The alkaline compound then removes carbon dioxide from the air “using the ocean as both reaction chamber and storage medium,” said Planetary Hydrogen CEO Mike Kelland. “Ultimately what the process does is produce carbon negative hydrogen.”
While this might require more energy per kg than other methods of producing green hydrogen, the added value of carbon removal can potentially make the price competitive. The process is a bit like direct-air capture of carbon dioxide, but Planetary Hydrogen cannot offer a stream of CO2 for sale or storage. Instead, its product is a carbon-negative hydrogen fuel, which the company expects will be attractive going forward given the growing interest in both clean energy and carbon extraction. The alkaline bicarbonate that results from the seawater carbon removal process also reduces ocean acidification.
Other approaches use heat or light, rather than electricity, to drive the chemical reactions. For instance, a thermochemical process applies high temperature heat from solar concentrators or nuclear reactors to water in the presence of other chemicals such as cerium oxide or copper chloride to split hydrogen from oxygen. The chemicals can be reused in a closed loop system that consumes only water.
Microorganisms such as green microalgae or cyanobacteria can split water into oxygen and hydrogen ions in the presence of sunlight in a process called photobiological water splitting. Researchers believe this approach can potentially be a low-cost, net-zero carbon process utilizing undrinkable water or wastewater, though this needs to be proven outside laboratory settings.
Download an Infographic: Clean Electricity Around the World
Finally, some researchers are trying to optimize photosynthetic hydrogen production by removing the biological limitations. Specialized semiconductors known as photoelectrochemical materials can split water in the presence of sunlight. The materials are similar to those used in photovoltaic cells but have been altered so they can be immersed in an electrolyte, where they facilitate what is called direct solar electrolysis or artificial photosynthesis. The approach, which is still at laboratory scale, can potentially provide a high-efficiency, low temperature process that could leverage the proliferation of solar PV technology to achieve low cost and scale.
According to Wipke, DOE’s NREL lab started out on a photoelectrochemical pathway—and reached efficiencies of 16 percent with that approach—but have now widened its focus to include biological approaches, a high-temperature approach utilizing both heat and electricity from a nuclear power plant, and the current front-runner: a low-temperature, electrolysis system based on proton exchange membranes and a polymer electrolyte similar to those used in fuel cells. They also have a power-to-gas pilot operational in California that utilizes excess renewable power to produce either hydrogen gas through electrolysis or renewable natural gas through a process of bio-methanation that uses microorganisms to add carbon dioxide to the hydrogen, producing renewable natural gas.
For green hydrogen to play a large part in decarbonizing the economy, one or more of these approaches—or something similar—will need a breakthrough on price, efficiency, and scalability.
That’s clearly DOE’s vision. The department’s Hydrogen Energy Earth Shot goal is to reduce the cost of green hydrogen by 80 percent to $1 per kilogram over the next 10 years—and expand its use to more than one-sixth of the United States’s primary energy use.
“This will enable a number of new markets including electric power generation, metals manufacturing, heavy transportation, and energy storage among others,” Wipke said, “where the use of clean hydrogen can add value on emissions reduction, economic and energy resilience fronts while being competitive with their more polluting predecessors.”
R.P. Siegel is a science and technology writer based in Rochester, N.Y.