Designing Better Concrete for SMRs
Designing Better Concrete for SMRs
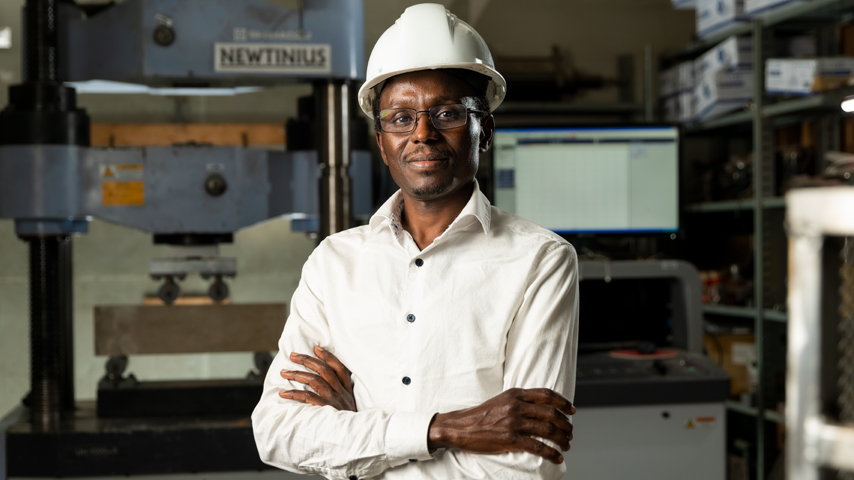
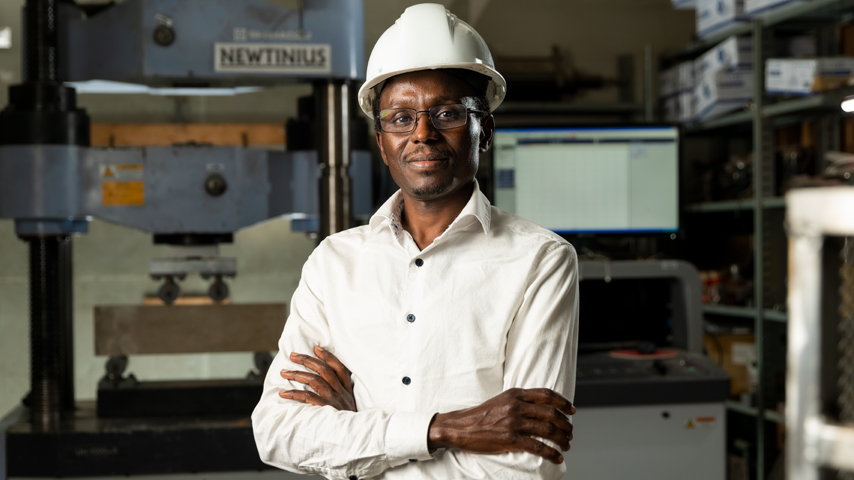
Researcher Ousmane Hisseine and colleagues are developing a stronger, denser material that can protect small modular reactors and the engineers who work on them.
Nuclear power has the potential to generate billions of kilowatt hours of electricity and do so without harmful greenhouse gas emissions. That promise has generated interest across the world and many countries are investing in new nuclear energy applications.
Today, countries like Canada, which struggle to supply power to remote areas in northern regions, are looking toward small modular reactors (SMRs), advanced nuclear reactors that can produce a large amount of low-carbon electricity. Such reactors require a smaller initial capital investment and can be deployed to a wide range of different sites, while their modular nature provides the opportunity for greater scalability by easily adding new reactors to the same power plant.
“These reactors are smaller in capacity and size than traditional nuclear reactors. They can be easily constructed in a modular process. The combination of the size and modularity make nuclear energy more affordable,” said Ousmane Hisseine, assistant professor of civil engineering at McMaster University in Hamilton, Ontario. “With the global drive toward decarbonization, these reactors can help move us toward cleaner energy.”
More on Advanced Nuclear Power: Nuclear Microreactor Nears Reality
Yet, it is imperative that engineers ensure nuclear reactors, even these SMRs, are safe for the communities they power. And that, Hisseine said, requires using the right materials to build both the SMRs and containment units for the waste they produce.
“Whenever anyone talks about nuclear energy, people’s first thoughts are about safety—and for good reason,” he said. “The majority of the physical infrastructure that provides safety to nuclear power plants relies on concrete. There is a significant need to create a better performing concrete that can address a variety of safety concerns.”
The dome that shelters a typical nuclear reactor is made of concrete and can be up to one meter thick. Nuclear waste is stored and transported in steel dry casks, which contain concrete inserts that are up to 75 centimeters thick to prevent the potentially hazardous contents from escaping.
“In comparison, the concrete wall we use in our homes is only about 20 centimeters thick,” Hisseine said. “We need the concrete in these domes and casks to be much thicker so we can capture the radiation inside and keep it from harming the plant workers or the outside environment.”
Hisseine and his colleagues are currently working on a new recipe for a novel concrete material specifically designed to improve safety in SMR nuclear facilities. The new material, called Damage-Tolerant Radiation-Shielding Ultra-High-Performance Concrete (DT-RS-UHPC), will be much stronger than typical concrete and will have a high energy absorption capacity.
Conventional concrete used for building has a strength measuring about 25-to-35 megapascals. The high-performance concrete used for bridges and other major infrastructure is stronger, in the 60-to-80 megapascal range. Hisseine’s goal is to create a new material with a strength of a whopping 120-to-150 megapascals.
“We want to double the strength of high-performance concrete so we can obtain higher resistance while remaining compact. We want to minimize any voids between ingredients to achieve higher radiation attenuation,” he explained. “With the right ingredients, we should also be able to achieve higher capacity, higher resistance, and higher durability to protect the concrete from radiation, damage, and deteriorative agents that may be outside the concrete containers.”
To support this work, Hisseine was one of four McMaster University scientists to receive a Small Modular Reactor research grant, funded by the Canadian Nuclear Safety Commission and the Natural Sciences and Engineering Research Council of Canada. Over the next two years, he and his colleagues hope to perfect the right combination of ingredients for this new concrete material with the power to help usher in a new generation of SMR-powered energy.
“The challenge we face is that some of the materials I will add to my concrete recipe to make the material resistant to radiation will negatively affect its resistance and strength. Other ingredients, including some type of Ironsand, which has a high iron content, may not perform as well at temperatures above 1,000 °C because the rocks inside it could expand in response to the higher temperature,” he said. “I need to optimize the dose of all the different attenuating materials to make sure the material is resistant to radiation, has the right strength, has high absorption to energy, and can work in elevated temperatures.”
While Hisseine knows it will be a lot of work—even once the recipe is complete, it will still require fine-tuning for specific SMR-related applications—he is optimistic that advances in concrete materials will help Canada move toward a cleaner energy economy.
“SMRs can offer off-grid energy sources to provide clean and reliable energy access to remote communities and in the mining sector,” he said. “With improved concrete materials, we can make nuclear energy safer and help this energy sector provide more and more clean energy across the country.”
Kayt Sukel is a technology writer in Houston.
Today, countries like Canada, which struggle to supply power to remote areas in northern regions, are looking toward small modular reactors (SMRs), advanced nuclear reactors that can produce a large amount of low-carbon electricity. Such reactors require a smaller initial capital investment and can be deployed to a wide range of different sites, while their modular nature provides the opportunity for greater scalability by easily adding new reactors to the same power plant.
“These reactors are smaller in capacity and size than traditional nuclear reactors. They can be easily constructed in a modular process. The combination of the size and modularity make nuclear energy more affordable,” said Ousmane Hisseine, assistant professor of civil engineering at McMaster University in Hamilton, Ontario. “With the global drive toward decarbonization, these reactors can help move us toward cleaner energy.”
More on Advanced Nuclear Power: Nuclear Microreactor Nears Reality
Yet, it is imperative that engineers ensure nuclear reactors, even these SMRs, are safe for the communities they power. And that, Hisseine said, requires using the right materials to build both the SMRs and containment units for the waste they produce.
“Whenever anyone talks about nuclear energy, people’s first thoughts are about safety—and for good reason,” he said. “The majority of the physical infrastructure that provides safety to nuclear power plants relies on concrete. There is a significant need to create a better performing concrete that can address a variety of safety concerns.”
The dome that shelters a typical nuclear reactor is made of concrete and can be up to one meter thick. Nuclear waste is stored and transported in steel dry casks, which contain concrete inserts that are up to 75 centimeters thick to prevent the potentially hazardous contents from escaping.
“In comparison, the concrete wall we use in our homes is only about 20 centimeters thick,” Hisseine said. “We need the concrete in these domes and casks to be much thicker so we can capture the radiation inside and keep it from harming the plant workers or the outside environment.”
Hisseine and his colleagues are currently working on a new recipe for a novel concrete material specifically designed to improve safety in SMR nuclear facilities. The new material, called Damage-Tolerant Radiation-Shielding Ultra-High-Performance Concrete (DT-RS-UHPC), will be much stronger than typical concrete and will have a high energy absorption capacity.
Conventional concrete used for building has a strength measuring about 25-to-35 megapascals. The high-performance concrete used for bridges and other major infrastructure is stronger, in the 60-to-80 megapascal range. Hisseine’s goal is to create a new material with a strength of a whopping 120-to-150 megapascals.
“We want to double the strength of high-performance concrete so we can obtain higher resistance while remaining compact. We want to minimize any voids between ingredients to achieve higher radiation attenuation,” he explained. “With the right ingredients, we should also be able to achieve higher capacity, higher resistance, and higher durability to protect the concrete from radiation, damage, and deteriorative agents that may be outside the concrete containers.”
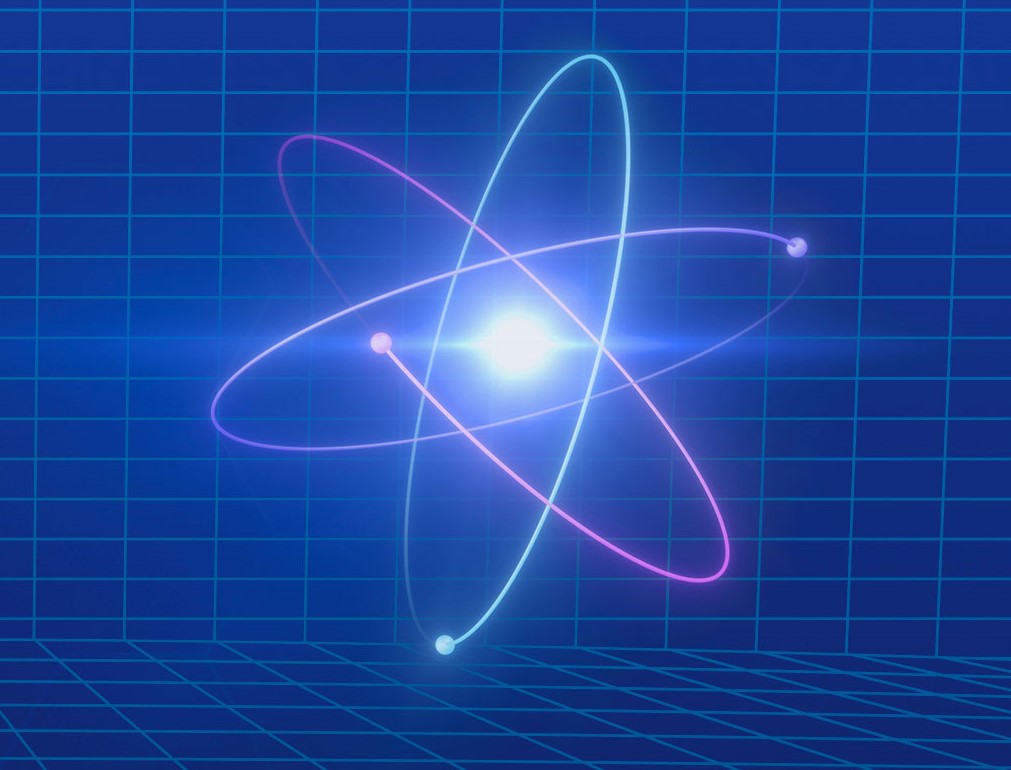
ASME's SMR and Advanced Reactor Portfolio
ASME continues to support the nuclear power industry.
“The challenge we face is that some of the materials I will add to my concrete recipe to make the material resistant to radiation will negatively affect its resistance and strength. Other ingredients, including some type of Ironsand, which has a high iron content, may not perform as well at temperatures above 1,000 °C because the rocks inside it could expand in response to the higher temperature,” he said. “I need to optimize the dose of all the different attenuating materials to make sure the material is resistant to radiation, has the right strength, has high absorption to energy, and can work in elevated temperatures.”
While Hisseine knows it will be a lot of work—even once the recipe is complete, it will still require fine-tuning for specific SMR-related applications—he is optimistic that advances in concrete materials will help Canada move toward a cleaner energy economy.
“SMRs can offer off-grid energy sources to provide clean and reliable energy access to remote communities and in the mining sector,” he said. “With improved concrete materials, we can make nuclear energy safer and help this energy sector provide more and more clean energy across the country.”
Kayt Sukel is a technology writer in Houston.