Designing a Golden Eye for the Sky
Designing a Golden Eye for the Sky
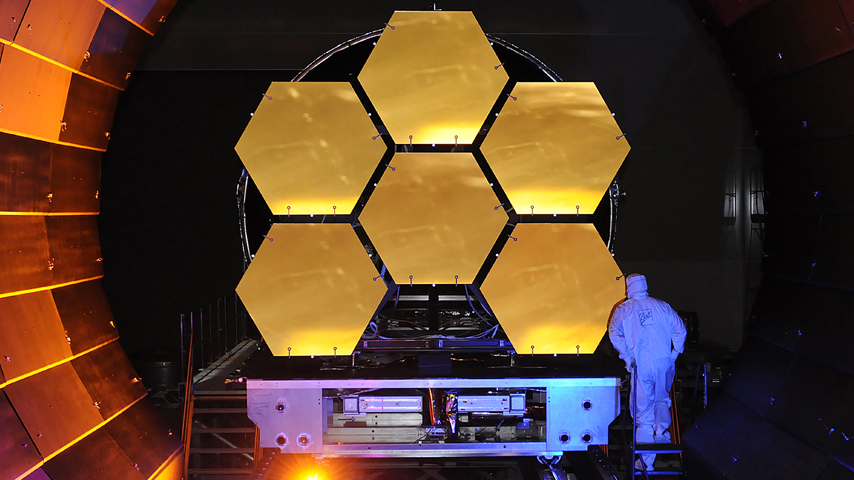
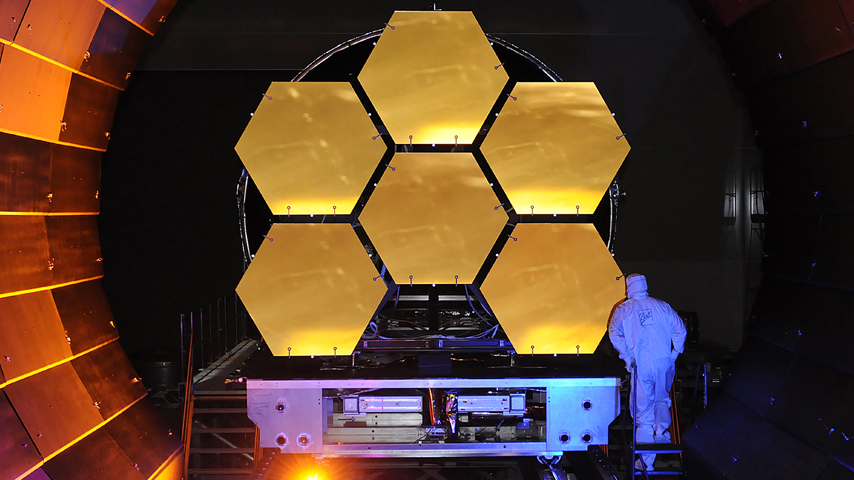
After 10 years and a $10-billion investment, the James Webb Space Telescope, NASA’s new optical telescope and its largest yet, is reshaping humanity’s understanding of the universe.
December 25, 2021, was far from an ordinary Christmas. At least not for several thousand NASA scientists and engineers who had been working nearly around the clock to prepare for the launch of the James Webb Space Telescope, NASA’s prized and sophisticated new eye in the sky.
The physical lift-off took place at the European Space Agency’s spaceport in Kourou, French Guiana, but NASA staff involved in the launch were stationed in numerous places. One of them was the Space Telescope Science Institute (STScI) in Maryland, which operates all NASA telescopes. Still deep in the throes of the pandemic, all employees seated at their computers in preparation for launch were wearing masks. Everyone was excited and anxious, with so much at stake.
“We rehearsed it a thousand times,” said Andrew Pedder, senior system engineer at STScI. “We rehearsed it to the point that everyone could have done it in their sleep, because of the number of times we practiced.”
The James Webb Telescope, commonly referred to as “The Observatory,” is NASA’s largest and most complex piece of astronomy equipment to date. Ten years in the making at a price tag of nearly $10 billion, Webb was designed to not only go where no other telescopes have gone before, but also see things no other telescopes have been able to see. Unlike the famous Hubble Telescope, Webb was going to peer billions of years back in time when the universe was first created after the Big Bang. To be able to do that, The Observatory’s construction was far different from its predecessors.
For starters, the telescope’s massive size meant it would not fit as is within a rocket. Instead, The Observatory was folded for take-off and later unfurled and assembled over time in space, all by itself—a feat of engineering that had never before been done.
Listen to an interview with the author: Behind the Webb
Because of its astronomical cost, NASA engineers were also unable to integrate the standard backup or redundancy systems typically found on comparable space equipment. As a result, even though there were more than 300 single points of failure that could happen along the way—of widely varying degrees, from reduced telescope ability to catastrophic failure—there would be no way to repair or correct them after launch.
Engineers designed Webb’s substantial size to ensure its ability to gather as much light from the universe as possible. A telescope’s sensitivity, or the amount of detail it can see, directly relates to the mirror size; the larger the mirror, the more light it collects. By comparison, the Hubble Space Telescope’s mirror measured 7.8 feet across, while Webb’s primary collecting mirror is nearly three times that at 21.7 feet. Webb’s primary mirror features even more light-collecting surface than Hubble’s 50 square feet, totaling 270 square feet. To transport the massive mirror into space, and to ensure it would even fit within a rocket, required a modular approach. Webb’s mirror is comprised of 18 hexagonal-shaped segments, each 4.3 feet in diameter and capable of folding and unfolding much like the wings of a dinner table.
Once gathered, light from the primary mirror is reflected onto Webb’s smaller secondary mirror, which is less than 2.5 feet in diameter. The secondary mirror then reflects light into multiple cameras that send images and data down to Earth through an antenna at the bottom of the telescope.
NASA’s team also had to solve a materials conundrum, as the mirror had to be both sturdy and relatively lightweight to make lift-off manageable. Moreover, the material needed the rare ability to behave in space the same way as it did on earth. Most metal objects would change their dimensional shape at least slightly, due to their typically significant coefficient of thermal expansion—a property that measures to what extent a material expands when heated and shrinks when cooled. Since the segmented mirror also needed to assemble itself, even the slightest change in shape caused by the cosmic cold could be disastrous.
Ultimately, the mirrors were made out of beryllium. “Not only is beryllium extremely lightweight, but it has the highest stiffness to weight ratio,” said Matthew Lallo, STScI systems scientist and manager of the telescopes branch. “Its strength-to-density ratio is four times better than steel or aluminum or titanium—it’s one of the stiffest, lightest metals.” Despite its size, the entire Webb Telescope weighs 14,000 pounds, compared to Hubble’s 27,000 pounds.
But the real magic of beryllium lies in its cryogenic properties. Unlike other metals, it doesn’t change shape with temperature fluxes, Lallo added. “Beryllium remains virtually unchanged dimensionally at any temperature. So you can cool it all the way down to absolute zero and it stays that shape, which is really unique among metals,” he explained. “All that made it an ideal choice for use in the Webb Telescope.”
One of The Observatory’s tasks is looking for different wavelengths of light than its predecessor Hubble, which was made to primarily see visible light, along with some ultraviolet and infrared. Webb was designed to detect the longer wavelengths of light in the infrared spectrum that faraway galaxies and stars emit. The telescope was specifically optimized for what astronomers call the red-shifting effect, which happens as light travels to Earth from far away, passing through the still-expanding universe. As the universe expands, which can be visualized as stretching, the light that travels through it gets stretched as well, so its wavelengths get longer, shifting from visible or ultraviolet lengths to longer lengths of infrared.
Discover the benefits of ASME membership
“The light emitted by faraway galaxies and stars arrives to us not as the visible light, but as the infrared,” said astronomer Nancy Levenson, STScI’s interim director. “And that’s what Webb is optimized to see.”
This meant Webb’s primary mirror needed the ability to reflect infrared light—something beryllium doesn’t do well. The solution was to coat the primary mirror in gold, which reflects about 99 percent of infrared light. To do that, all 18 mirror segments were placed inside a vacuum chamber, where a small amount of gold was vaporized and deposited onto their surfaces in 100-nanometer-thick layers.
To complete the manufacturing process, Webb’s 18 mirror segments traveled across the country: Beryllium powder from Utah was formed into mirrors, coatings were applied at Ball Aerospace & Technologies Corp. in Boulder, Colo., and the gold layer was applied at Quantum Coating, Inc., in Moorestown, N.J. In total, the telescope made 14 stops in 11 different places before finally arriving at Kourou as one “Golden Eye,” ready for its long space journey.
Webb has gone where no other telescopes have gone before—it had a much longer way to travel too. The Ariane 5 rocket’s takeoff was only the beginning of a roughly month-long journey. Unlike Hubble, which orbits the Earth right above the atmosphere, about 332 miles up, Webb is stationed roughly 1 million miles out in space at the second Lagrange point. These are space “parking spots,” where the gravitational pull of celestial bodies like the sun and Earth equalize just enough so that an object can circle around them nearly forever with minimal use of fuel to correct its course. “It’s doing a little orbit around that particular point, even though there’s nothing there to orbit around,” Levenson said, noting that gravitational forces will keep the telescope circling.
During its odyssey, Webb went through a few important assembly milestones. Half an hour after launch, the telescope’s system established its connection with STScI. On the third day of its voyage, preparations began to unwrap the sunshield—a five-layer parasol the size of a tennis court that was built to protect the telescope from the heat emitted by the sun or reflected by other celestial bodies.
Since the Webb Telescope gathers infrared light, which is essentially heat, the mirrors must be kept cold for proper data collection. Built from Kapton, a lightweight material that tolerates both extreme heat and extreme cold, the shield would keep the observatory at 40 K or -388 ºF. Some parts of Webb’s equipment are kept even colder, below 7 K, by pumping warmth-absorbing helium through its instruments.
Six days into the journey, mid-booms deployed to expand the Kapton parasol’s five layers. Ten days past launch, the secondary mirror extended. On days 12 and 13, Webb spread its wings by opening the mirror components that were folded for takeoff.
Now that Webb was assembled, each of the 18 mirror segments had to be fine-tuned so that they would gather and reflect light properly onto the secondary mirror. This was crucial to receiving a clear picture of anything The Observatory might detect. When reflected onto the secondary mirror, light waves are supposed to amplify each other, Lallo explained.
“You have to have the wavelengths of light to constructively interfere instead of destructively interfere, which we call ‘phasing the wavefront,’” he said. “If one wave arrives at its trough when another one arrives at its peak, they sort of cancel out.” Therefore, the waves from each of the 18 segments must bounce off the secondary mirror and hit the camera not just at the same place, but at the same point in the wave.
Watch our video on the Webb Telescope
To achieve such precision, each of Webb’s 18 mirror segments are controlled by cryogenic actuators, designed to not only function in the extreme cold, but also to adjust the segments at two different levels: coarse, at the centimeter scale, and fine, at the nanometer scale. Coarse adjustments helped align the segments after the mirror was first assembled in space. Fine adjustments are necessary for experiments when researchers would like to view specific objects. A remote-controlled pin can switch the actuators between their coarse and fine modes.
When Webb finally completed its unfolding sequence and parked at the Lagrange point, the image sharpness that the telescope began sending back to Earth exceeded all expectations. Once all the segments were aligned and fine-tuned, the image quality was superb.
“We were amazed at how sharp the images were,” Levenson said, adding that years of hard work paid off almost immediately. “People are already detecting the stars and galaxies from about 300 million years after the Big Bang. That sounds like a long time but considering that the Big Bang happened 13.7 billion years ago, that’s very close to the beginning. So these really are some of the earliest galaxies that we’re detecting.”
Some new discoveries may even reshape humanity’s understanding of how the universe was created and how stars formed. In summer 2022, a group of American and European researchers found several unusually bright red spots on Webb images, not spotted by Hubble because it can only detect a limited amount of infrared light. The team believes that the spots are six huge galaxies with millions of stars, all created very early in cosmic time, about 500 to 700 million years after the Big Bang. However, according to the current Big Bang postulates, this isn’t enough time to create so many massive galaxies with so many stars.
Engineering in orbit: Space Station Could Bring Weight to the Weightless
“According to our current theories, it should take much longer for galaxies of this mass to be able to form,” said Erica Nelson, researcher at the Colorado State University’s Astrophysical and Planetary Sciences and co-author of “A population of red candidate massive galaxies ~600 Myr after the Big Bang,” published in Nature in April 2023. “So we may need to make some kind of modifications to our cosmological model.”
Webb will also help astronomers check for signs of biological activities in the atmospheres of planets in other solar systems, Levenson added. But to effectively search for life on those planets, NASA would have to build another observatory optimized specifically for that task. “So, we actually need the next telescope to be able to do that,” Levenson said. “And it would have to be even bigger than Webb.”
Lina Zeldovich is a science writer in Woodside, N.Y.
The physical lift-off took place at the European Space Agency’s spaceport in Kourou, French Guiana, but NASA staff involved in the launch were stationed in numerous places. One of them was the Space Telescope Science Institute (STScI) in Maryland, which operates all NASA telescopes. Still deep in the throes of the pandemic, all employees seated at their computers in preparation for launch were wearing masks. Everyone was excited and anxious, with so much at stake.
“We rehearsed it a thousand times,” said Andrew Pedder, senior system engineer at STScI. “We rehearsed it to the point that everyone could have done it in their sleep, because of the number of times we practiced.”
The James Webb Telescope, commonly referred to as “The Observatory,” is NASA’s largest and most complex piece of astronomy equipment to date. Ten years in the making at a price tag of nearly $10 billion, Webb was designed to not only go where no other telescopes have gone before, but also see things no other telescopes have been able to see. Unlike the famous Hubble Telescope, Webb was going to peer billions of years back in time when the universe was first created after the Big Bang. To be able to do that, The Observatory’s construction was far different from its predecessors.
For starters, the telescope’s massive size meant it would not fit as is within a rocket. Instead, The Observatory was folded for take-off and later unfurled and assembled over time in space, all by itself—a feat of engineering that had never before been done.
Listen to an interview with the author: Behind the Webb
Because of its astronomical cost, NASA engineers were also unable to integrate the standard backup or redundancy systems typically found on comparable space equipment. As a result, even though there were more than 300 single points of failure that could happen along the way—of widely varying degrees, from reduced telescope ability to catastrophic failure—there would be no way to repair or correct them after launch.
Finding the light
Engineers designed Webb’s substantial size to ensure its ability to gather as much light from the universe as possible. A telescope’s sensitivity, or the amount of detail it can see, directly relates to the mirror size; the larger the mirror, the more light it collects. By comparison, the Hubble Space Telescope’s mirror measured 7.8 feet across, while Webb’s primary collecting mirror is nearly three times that at 21.7 feet. Webb’s primary mirror features even more light-collecting surface than Hubble’s 50 square feet, totaling 270 square feet. To transport the massive mirror into space, and to ensure it would even fit within a rocket, required a modular approach. Webb’s mirror is comprised of 18 hexagonal-shaped segments, each 4.3 feet in diameter and capable of folding and unfolding much like the wings of a dinner table.
Once gathered, light from the primary mirror is reflected onto Webb’s smaller secondary mirror, which is less than 2.5 feet in diameter. The secondary mirror then reflects light into multiple cameras that send images and data down to Earth through an antenna at the bottom of the telescope.
NASA’s team also had to solve a materials conundrum, as the mirror had to be both sturdy and relatively lightweight to make lift-off manageable. Moreover, the material needed the rare ability to behave in space the same way as it did on earth. Most metal objects would change their dimensional shape at least slightly, due to their typically significant coefficient of thermal expansion—a property that measures to what extent a material expands when heated and shrinks when cooled. Since the segmented mirror also needed to assemble itself, even the slightest change in shape caused by the cosmic cold could be disastrous.
Ultimately, the mirrors were made out of beryllium. “Not only is beryllium extremely lightweight, but it has the highest stiffness to weight ratio,” said Matthew Lallo, STScI systems scientist and manager of the telescopes branch. “Its strength-to-density ratio is four times better than steel or aluminum or titanium—it’s one of the stiffest, lightest metals.” Despite its size, the entire Webb Telescope weighs 14,000 pounds, compared to Hubble’s 27,000 pounds.
But the real magic of beryllium lies in its cryogenic properties. Unlike other metals, it doesn’t change shape with temperature fluxes, Lallo added. “Beryllium remains virtually unchanged dimensionally at any temperature. So you can cool it all the way down to absolute zero and it stays that shape, which is really unique among metals,” he explained. “All that made it an ideal choice for use in the Webb Telescope.”
One of The Observatory’s tasks is looking for different wavelengths of light than its predecessor Hubble, which was made to primarily see visible light, along with some ultraviolet and infrared. Webb was designed to detect the longer wavelengths of light in the infrared spectrum that faraway galaxies and stars emit. The telescope was specifically optimized for what astronomers call the red-shifting effect, which happens as light travels to Earth from far away, passing through the still-expanding universe. As the universe expands, which can be visualized as stretching, the light that travels through it gets stretched as well, so its wavelengths get longer, shifting from visible or ultraviolet lengths to longer lengths of infrared.
Discover the benefits of ASME membership
“The light emitted by faraway galaxies and stars arrives to us not as the visible light, but as the infrared,” said astronomer Nancy Levenson, STScI’s interim director. “And that’s what Webb is optimized to see.”
This meant Webb’s primary mirror needed the ability to reflect infrared light—something beryllium doesn’t do well. The solution was to coat the primary mirror in gold, which reflects about 99 percent of infrared light. To do that, all 18 mirror segments were placed inside a vacuum chamber, where a small amount of gold was vaporized and deposited onto their surfaces in 100-nanometer-thick layers.
To complete the manufacturing process, Webb’s 18 mirror segments traveled across the country: Beryllium powder from Utah was formed into mirrors, coatings were applied at Ball Aerospace & Technologies Corp. in Boulder, Colo., and the gold layer was applied at Quantum Coating, Inc., in Moorestown, N.J. In total, the telescope made 14 stops in 11 different places before finally arriving at Kourou as one “Golden Eye,” ready for its long space journey.
The space and assembly odyssey
Webb has gone where no other telescopes have gone before—it had a much longer way to travel too. The Ariane 5 rocket’s takeoff was only the beginning of a roughly month-long journey. Unlike Hubble, which orbits the Earth right above the atmosphere, about 332 miles up, Webb is stationed roughly 1 million miles out in space at the second Lagrange point. These are space “parking spots,” where the gravitational pull of celestial bodies like the sun and Earth equalize just enough so that an object can circle around them nearly forever with minimal use of fuel to correct its course. “It’s doing a little orbit around that particular point, even though there’s nothing there to orbit around,” Levenson said, noting that gravitational forces will keep the telescope circling.
During its odyssey, Webb went through a few important assembly milestones. Half an hour after launch, the telescope’s system established its connection with STScI. On the third day of its voyage, preparations began to unwrap the sunshield—a five-layer parasol the size of a tennis court that was built to protect the telescope from the heat emitted by the sun or reflected by other celestial bodies.
Since the Webb Telescope gathers infrared light, which is essentially heat, the mirrors must be kept cold for proper data collection. Built from Kapton, a lightweight material that tolerates both extreme heat and extreme cold, the shield would keep the observatory at 40 K or -388 ºF. Some parts of Webb’s equipment are kept even colder, below 7 K, by pumping warmth-absorbing helium through its instruments.
Six days into the journey, mid-booms deployed to expand the Kapton parasol’s five layers. Ten days past launch, the secondary mirror extended. On days 12 and 13, Webb spread its wings by opening the mirror components that were folded for takeoff.
Now that Webb was assembled, each of the 18 mirror segments had to be fine-tuned so that they would gather and reflect light properly onto the secondary mirror. This was crucial to receiving a clear picture of anything The Observatory might detect. When reflected onto the secondary mirror, light waves are supposed to amplify each other, Lallo explained.
“You have to have the wavelengths of light to constructively interfere instead of destructively interfere, which we call ‘phasing the wavefront,’” he said. “If one wave arrives at its trough when another one arrives at its peak, they sort of cancel out.” Therefore, the waves from each of the 18 segments must bounce off the secondary mirror and hit the camera not just at the same place, but at the same point in the wave.
Watch our video on the Webb Telescope
To achieve such precision, each of Webb’s 18 mirror segments are controlled by cryogenic actuators, designed to not only function in the extreme cold, but also to adjust the segments at two different levels: coarse, at the centimeter scale, and fine, at the nanometer scale. Coarse adjustments helped align the segments after the mirror was first assembled in space. Fine adjustments are necessary for experiments when researchers would like to view specific objects. A remote-controlled pin can switch the actuators between their coarse and fine modes.
When Webb finally completed its unfolding sequence and parked at the Lagrange point, the image sharpness that the telescope began sending back to Earth exceeded all expectations. Once all the segments were aligned and fine-tuned, the image quality was superb.
“We were amazed at how sharp the images were,” Levenson said, adding that years of hard work paid off almost immediately. “People are already detecting the stars and galaxies from about 300 million years after the Big Bang. That sounds like a long time but considering that the Big Bang happened 13.7 billion years ago, that’s very close to the beginning. So these really are some of the earliest galaxies that we’re detecting.”
Some new discoveries may even reshape humanity’s understanding of how the universe was created and how stars formed. In summer 2022, a group of American and European researchers found several unusually bright red spots on Webb images, not spotted by Hubble because it can only detect a limited amount of infrared light. The team believes that the spots are six huge galaxies with millions of stars, all created very early in cosmic time, about 500 to 700 million years after the Big Bang. However, according to the current Big Bang postulates, this isn’t enough time to create so many massive galaxies with so many stars.
Engineering in orbit: Space Station Could Bring Weight to the Weightless
“According to our current theories, it should take much longer for galaxies of this mass to be able to form,” said Erica Nelson, researcher at the Colorado State University’s Astrophysical and Planetary Sciences and co-author of “A population of red candidate massive galaxies ~600 Myr after the Big Bang,” published in Nature in April 2023. “So we may need to make some kind of modifications to our cosmological model.”
Webb will also help astronomers check for signs of biological activities in the atmospheres of planets in other solar systems, Levenson added. But to effectively search for life on those planets, NASA would have to build another observatory optimized specifically for that task. “So, we actually need the next telescope to be able to do that,” Levenson said. “And it would have to be even bigger than Webb.”
Lina Zeldovich is a science writer in Woodside, N.Y.